A large-scale, real-life experiment
continues to be run. The "piston effect",
as described elsewhere on these web pages, makes it more difficult
to attain the 0.07 ppm 8-hour ozone standard compared to the
0.075 ppm standard. The piston effect says that as ozone control
strategies are implemented, the higher concentrations decrease
faster than the concentrations below the highest values. In fact,
the distribution of hourly ozone concentrations at the lower
level actually increase at some locations. The result of the
"piston effect" is that as
new control strategies are implemented, the states, tribes, and
the US EPA will see some progress in the reduction of hourly
average ozone concentrations at sites that experience 0.10 ppm
and above. These reductions will translate into lower 4th highest
seasonal 8-hour daily maximum concentrations. As the states,
tribes, and the EPA continue to strive for further reductions
in the 8-hour average concentrations by attempting to reduce
the hourly average concentrations below 0.09 ppm, because of
the "piston" effect, progress will begin to slow down.
The EPA, tribes, and the states may notice that the implemented
control strategies may not be working as effectively as models
originally predicted and, in some cases, governmental entities
may conclude erroneously that more stringent local controls may
be needed. In some locations, the 8-hour standard will be attained
for specific years but in other years, the 8-hour standard may
be violated at the same location. As the years go by, an oscillation
in and out of violation may occur. Please visit our concerns
web page to learn more about the piston effect and how it may
be influencing the U.S. ozone trends that have occurred over
the years. On that page, please note the lower trending rate
in the 8-hour ozone concentrations nationwide in the shorter
trending period compared to the longer trending period.
Research reported in the literature has
described the problems in reducing hourly concentrations of ozone.
Lefohn et al. (1998) identified those sites that demonstrated
a significant reduction in ozone levels for the period 1980-1995.
Using the data from the sites that experienced reduced ozone
levels over the period of time, the authors investigated whether
the rate of decline of the mid-level hourly average concentrations
was similar to the rate experienced by the high hourly average
concentrations. The analysis indicated that there is a greater
resistance to reducing the hourly average concentrations in the
mid range than the hourly average concentrations above 0.09 ppm.
Figure 1 below is an example that shows that the higher hourly
average concentrations (i.e., above 90 ppb) decreased at a faster
rate (greater negative rate per year) than the hourly average
concentrations in the mid-level range. The numbers of hourly
average concentrations in the low end of the distribution also
decreased (i.e., the concentrations in the low end of the distribution
moved upwards). Both the high and low ends of the distribution
moved toward the center of the distribution.
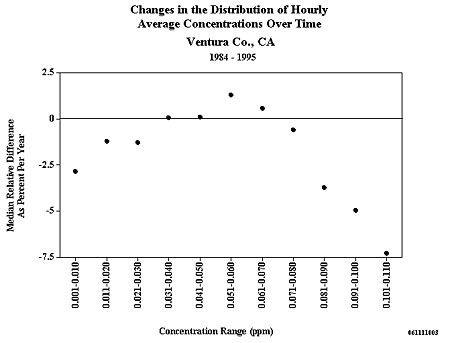
Figure 1
Lefohn et al. (1998) discussed the movement
of the low hourly average ozone concentrations toward the mid-level
values (i.e., the decrease of the frequency of the lower hourly
average concentrations). Figure 2 illustrates the frequency of
occurrence
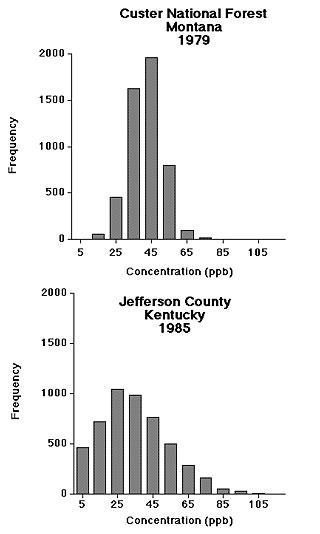
Figure 2
of hourly average ozone concentrations
at two monitoring sites. The Custer National Forest site in Montana
experiences very low maximum hourly average concentrations. The
distribution of the hourly average concentrations at the site
shows a lack of both high and low hourly average concentrations.
The number of lower hourly average concentrations is reduced
because of a lack of NOx scavenging. As reductions in emissions
occur, the shift from the lower hourly average concentrations
to mid-level values is expected to occur. For 1980, more than
10% of the hourly average and 8-hour daily maximum concentrations
at the site were above 0.05 and 0.059 ppm, respectively. The
hourly and 8-hour daily maximum concentrations above 0.05 ppm
at this site may not be associated with long-range transport
of ozone and its precursors from more polluted locations. The
site experienced its highest hourly average concentrations in
April and May; this is when most sites in the United States do
not experience high hourly average average concentrations. This,
coupled with the observation that the diurnal maximum concentrations
occurred between 1400 and 1500 local time, implies that the ozone
may have been generated locally or meteorological processes are
transporting the ozone down from aloft. The sources for creating
the ozone may have been natural (e.g., stratospheric intrustions).
The distribution pattern of the hourly
average concentrations for a heavily urban-influenced monitoring
site at Jefferson County, Kentucky is shown in Figure 2. In contrast
to the rural site in Montana, the urban-influenced site in Kentucky
showed frequent high and low hourly average concentrations. This
site appeared to be influenced by NOx scavenging because of the
occurrence of more frequent low hourly average concentrations.
Lefohn et al. (1998) reported in their
trends analyses, that as ozone levels improved for several urban
sites, both the high and the low hourly average concentrations
moved toward the 0.03-0.06 ppm range, which is within the range
of concentrations that most frequently occurred at the rural
site in Montana. Lefohn et al. (1998) hypothesized that as adequate
control strategies were implemented, the distribution pattern
of hourly average concentrations for inland monitoring sites
would approach the pattern observed at the Montana site and other
remote sites in the western United States.
Coyle, Fowler, and Ashmore (2003) have
reported for an analysis of United Kingdom monitoring data that
peak ozone concentrations declined by about 30% over the past
decade, but that there was evidence of an increase in annual
mean concentrations of about 0.1 ppb per year. Using simulation
modeling, the authors reported that the lower concentrations
increased. Although the authors hypothesize that this increase
may reflect the impact of global increases in background concentrations,
such is not the case. As emissions reductions occur, at many
sites there are increases
in ozone concentrations at the lower levels, which is consistent
with data obtained in both the US and Europe (Lefohn et al.,
2017; Lefohn et al., 2018). Simon et al. (2015) reported similar
results for data in the U.S. This is mostly associated with reduced
titration of ozone by reaction with NO in response to reductions
in NOx emissions (see Lefohn et al., 2017). The shifting of the
lower concentrations toward the mid values results in many cases
with the median and annual average concentrations increasing
(see Lefohn et al., 2017). The use of annual average and median
ozone concentrations in global modeling for validation purposes
obfuscates the validation of the models due to the "shifting"
effects associated with the reduction of NOx scavenging. Tørseth et al. (2012) cautioned that the use
of annual mean values is of little help for evaluating ozone
trends due to the shifting effects associated with the low end
coming up and the high end coming down of the ozone distribution.
Reduced NOx emissions will give rise to a narrower frequency
distribution of ozone. The number of both the low and the high
ozone concentrations should go down as explained above if the
NOx emissions are reduced (i.e., a compression of the distribution
of the hourly average ozone concentrations). One might assume
that emissions reductions would result in lower ozone concentrations,
but such does not occur when focusing on median and annual average
concentrations. Van Malderen
(2021) notes that the interpretation of increasing mean surface
ozone concentrations is hampered by the interplay of many factors,
such as (1) meteorology and transport, (2) the non-linear dependence
of the ozone concentrations on the emissions of VOC and NOx,
(3) the dual role of NOx as an ozone source or sink depending
on the season, and (4) the amount of NOx emissions. Recently,
the IPCC AR6 Report (2021) noted that the decrease of NO emissions
in specific highly polluted areas can lead to the observed increase
in surface ozone concentrations in cities. In conclusion, the
use of median and
annual average ozone concentrations as exposure metrics to assess
current and future possible ozone effects on human health and
vegetation is highly questionable. Further discussion of the
limitation of the use of median and annual average ozone concentrations
for assessing effects on human health and vegetation can be found
in Lefohn et al. (2017), Lefohn et al. (2018), and Lefohn (2023).
As noted above, Lefohn et al. (1998) reported
decreases in the frequency of the lowest ozone concentrations
and increases in the mid-level concentrations and believed that
the decreases in frequency at the lower concentrations were due
to reduced NOx scavenging. In addition, (as noted here
and here)
no changes have been observed in the 4th highest 8-hour concentration
at some remote and relatively remote clean national park sites
in the United States. Lefohn et al. (2017) (please see publications list for additional references)
also noted that some sites in the western US experienced no trends
in surface ozone concentrations using several exposure indices
related to human health and vegetation.
The "piston effect" is real and
it appears that the implementation of politically acceptable
control strategies may never be able to allow many violating
areas to reach attainment on a continuous basis when the
standard is lowered to lower and lower levels. Some nonattainment
areas will continue to oscillate into and out of violation. Nature
has provided us with the "piston effect" and the challenge
is how best to work with it. Our research continues on the "piston
effect".
In assessing the efficacy of air pollution
reduction programs, it is important to determine whether 1) expected
emission reductions have occurred, 2) actual emission changes
resulted in changes in ambient concentrations consistent with
the predictions of air quality models, 3) changes in ambient
concentrations have resulted in reductions in human and ecosystem
exposure to the air pollutants in question, and 4) reductions
have led to improved public health and reduced damage to sensitive
ecosystems. If inconsistent observations are found to occur,
then it is quite possible that there were problems with the assumptions
used in the development of the 8-hour standard. If so, it will
be necessary to assess the physical, biological, and mathematical
methodologies used to develop the ozone standard prior to reaching
the simple conclusion that more emission reductions are needed.
It is important to better understand the physical and biological
processes at work. The answer
may not lie necessarily in more stringent emission controls,
but in assessing whether the form of the 8-hour average standard
could be replaced with a different form that will provide for
the same amount of human health protection and yet be attainable. A.S.L. & Associates is actively performing research
in this area.
References
Coyle M., Fowler, D. and
Ashmore M. (2003) New Directions: Implications of Increasing
Tropospheric Background Ozone Concentrations for Vegetation.
Atmospheric Environment. 37:153-154.
IPCC, 2021: Climate Change
2021: The Physical Science Basis. Contribution of Working Group
I to the Sixth Assessment Report of the Intergovernmental Panel
on Climate Change [Masson-Delmotte, V., P. Zhai, A. Pirani, S.
L. Connors, C. Péan, S. Berger, N. Caud, Y. Chen, L. Goldfarb,
M. I. Gomis, M. Huang, K. Leitzell, E. Lonnoy, J. B. R. Matthews,
T. K. Maycock, T. Waterfield, O. Yelekçi, R. Yu and B.
Zhou (eds.)]. Cambridge University Press. In Press.
Lefohn A. S., Shadwick
D. S. and Ziman S. D., 1998. The Difficult Challenge of Attaining
EPA's New Ozone Standard. Environmental Science & Technology.
32(11):276A-282A.
Lefohn, A.S., Malley, C.S.,
Simon, H., Wells. B., Xu, X., Zhang, L., Wang, T., 2017. Responses
of human health and vegetation exposure metrics to changes in
ozone concentration distributions in the European Union, United
States, and China. Atmospheric Environment 152: 123-145. doi:10.1016/j.atmosenv.2016.12.025.
Lefohn, A.S., Malley, C.S.,
Smith, L., Wells, B., Hazucha, M., Simon, H., Naik, V., Mills,
G., Schultz, M.G., Paoletti, E., De Marco, A., Xu, X., Zhang,
L., Wang, T., Neufeld, H.S., Musselman, R.C., Tarasick, T., Brauer,
M., Feng, Z., Tang, T., Kobayashi, K., Sicard, P., Solberg, S.,
and Gerosa. G., 2018. Tropospheric ozone assessment report: global
ozone metrics for climate change, human health, and crop/ecosystem
research. Elem Sci Anth. 2018;6(1):28. DOI:
http://doi.org/10.1525/elementa.279.
Lefohn, A.S. (2023). Comments on the Policy
Assessment for the Reconsideration of the Ozone National Ambient
Air Quality Standards, Draft Version 2 - Docket ID No. EPA-HQ-OAR-2018-0279-0617.
Simon H, Reff A, Wells B, Xing J, Frank
N. 2015. Ozone trends across the United States over a period
of decreasing NOx and VOC emissions. Environ Sci Technol 49:
186-195. dx.doi.org/10.1021/es504514z.
Tørseth, K., Aas,
W., Breivik, K., Fjæraa, A.M., Fiebig, M., Hjellbrekke,
A.G., Lund Myhre, C., Solberg, S., and Yttri, K.E., 2012. Introduction
to the European Monitoring and Evaluation Programme (EMEP) and
observed atmospheric composition change during 19722009.
Atmos. Chem. Phys., 12, 54475481, 2012
www.atmos-chem-phys.net/12/5447/2012/doi:10.5194/acp-12-5447-2012.
Van Malderen, R., De Muer,
D., De Backer, H., Poyraz, D., Verstraeten, W.W., De Bock, V.,
Delcloo, A.W., Mangold, A., Laffineur, Q., Allaart, M., Fierens,
F., and Thouret, V., 2021. Fifty years of balloon-borne ozone
profile measurements at Uccle,
Belgium: a short history, the scientific relevance, and the achievements
in understanding the vertical ozone distribution. Atmos. Chem.
Phys., 21, 1238512411, 2021. https://doi.org/10.5194/acp-21-12385-2021.